23.11.2023 / 09:00
Intercontinental Transport of Hydrogen and its Derivatives
Energy Markets
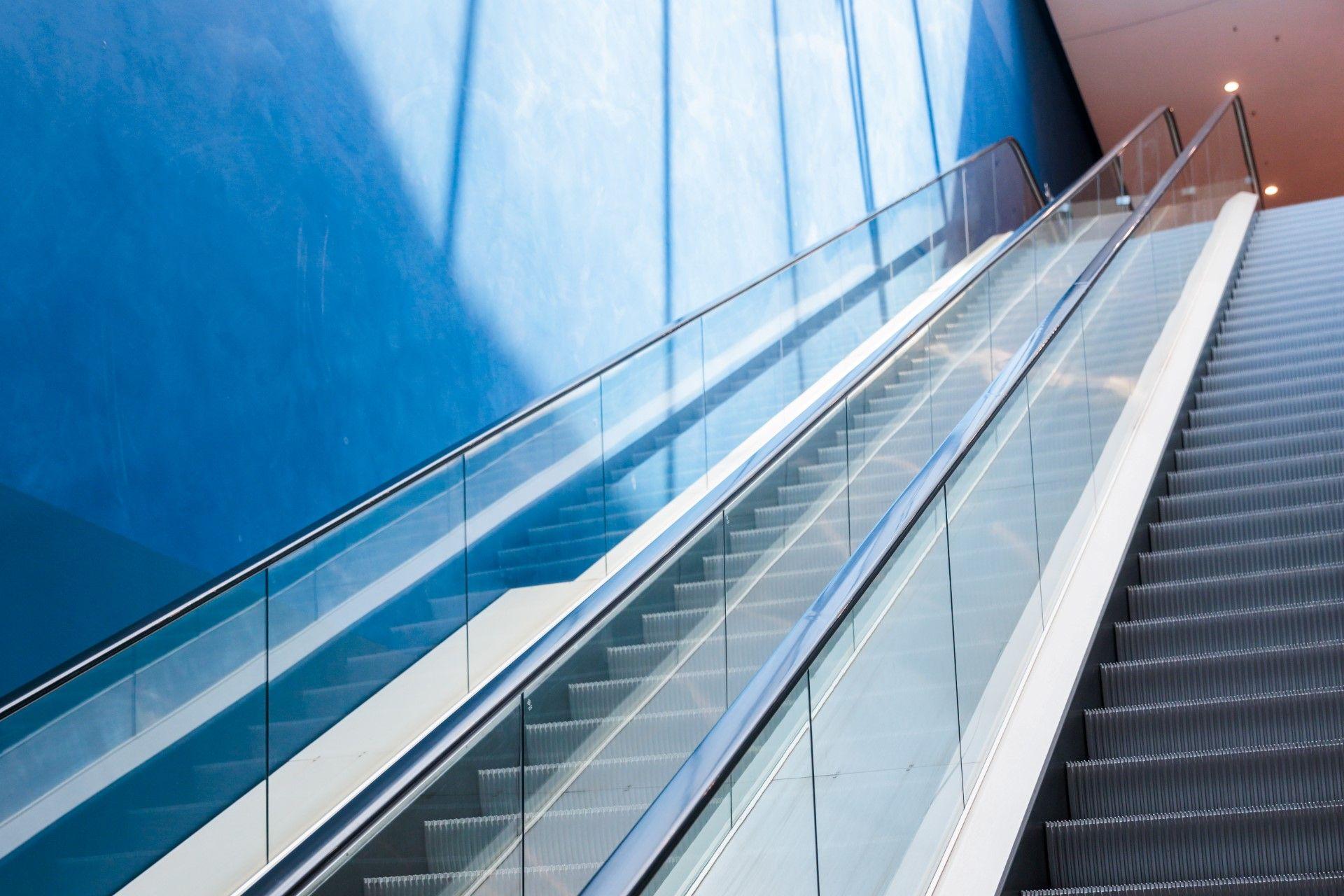
Green hydrogen is anticipated to play a key role in Germany’s goal of climate neutrality by 2045. To this effect, Germany‘s updated National Hydrogen Strategy sets a target of installing 10 GW of domestic electrolysis capacity by 2030. This would enable the production of approximately one million metric tonnes of hydrogen annually. However, it is anticipated that Germany’s domestic production capabilities would only satisfy approximately 26-35% (about 33TWh) of the country‘s hydrogen demand for 2030 (95-130 TWh).
Therefore, hydrogen imports will play an important role in meeting Germany’s domestic demand. For short-distance imports, several studies have suggested that pipelines are likely to be the most cost-effective mode of hydrogen transport. This, however, significantly limits the viable market area. For truly long-distance – i.e. intercontinental transportation - shipping is most viable. The most feasible current options for shipping hydrogen are through direct liquefication at cryogenic temperatures (LH2), or by transformation of hydrogen into a hydrogen carrier, of which (green) ammonia (NH3) shows the most promise. Furthermore, given that the cost of renewable electricity constitutes the most significant portion of the levelized cost of energy for green hydrogen, Germany will seek to source hydrogen from nations abundant in green energy resources. In pursuit of this goal, Germany has forged energy partnerships with the aim of cost-effective green hydrogen imports from nations such as Australia, Chile, Namibia, Saudi Arabia, and Canada.
Physical Characteristics of Liquid Hydrogen and Ammonia
The most important physical properties of Hydrogen and Ammonia determining their viability for long-distance transportation via ship can be summarized as follows:
Gravimetric and volumetric energy densities:
Gravimetric and volumetric energy densities measure energy per weight and volume respectively and are essential for ship-based energy storage and transport. For ship-based energy transport and storage, volumetric density is of greater importance.
Boil-off gas (BOG) losses:
BOG losses occur when heat transfers to the storage tank with liquefied hydrogen or ammonia. This heat can cause some stored hydrogen to vaporize, leading to high tank pressures. To alleviate this, the gas inside the storage is vented outside, resulting in boil-off gases. Such losses occur during intermediate storage, transportation, and the loading/unloading process.
Conversion & regasification losses:
There are several methodologies for the liquification of LH2 and NH3 and the reconversion to their gaseous states. Such methodologies are all highly energy-intensive processes. Additionally, the dehydrogenation process, extracting hydrogen from ammonia, incurs losses during reconversion. Such losses can however be avoided by directly using NH3 in specific industrial and agricultural applications, thus saving energy.
These properties are summarized for LH2, NH3 in the Figure below. For comparison, LNG, which is currently the most economically viable and widely used long-distance energy carrier, is also included.
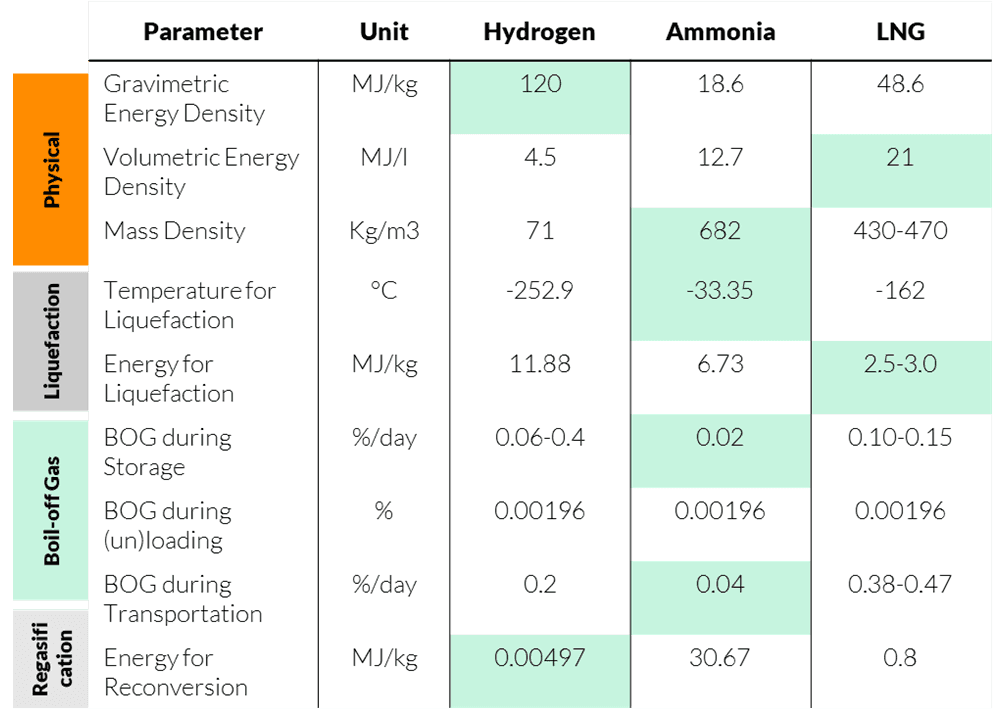
Analysis
Liquid Hydrogen:
Liquid hydrogen boasts an exceptional gravimetric energy density and requires insignificant amounts of energy for regassification. In terms of the other parameters considered, it is clearly at a disadvantage both to ammonia and LNG.
LNG:
Traditional LNG offers the optimal volumetric energy density and requires (comparatively) little energy for liquification. It however falls short compared to ammonia in terms of temperature requirements, and overall BOG performance. Despite producing lower CO2 emissions than traditional marine fuels, LNG is not considered a carbon-neutral fuel, making its environmental impact a significant consideration in the context of the shipping industry‘s increasing focus on sustainability.
Ammonia:
Ammonia‘s volumetric energy density, though less than that of LNG, still significantly exceeds that of LH2. Coupled with its favorable mass density and temperature requirements, Ammonia emerges as a highly practical choice as a low carbon energy carrier. Ammonia has superior BOG loss ratios during both storage and transportation, contributing to its overall suitability. Although ammonia‘s regasification energy requirements are higher, it becomes a compelling option, especially when used directly
Conclusion
In conclusion, Germany‘s ambitious hydrogen strategy underscores the nation‘s commitment to integrating green hydrogen into its energy landscape. While the target of 10 GW domestic electrolysis capacity by 2030 is a significant step, the projections reveal a substantial gap between domestic production and anticipated demand, necessitating a reliance on hydrogen imports. The strategic approach of sourcing from nations with competitive production costs, such as Australia, Chile, Namibia, Saudi Arabia, and Canada, aligns with Germany‘s emphasis on cost-effective green hydrogen. The analysis of long-distance transportation options emphasizes the importance of choosing between liquid hydrogen and ammonia, with ammonia emerging as a promising choice given its favorable physical characteristics, including superior volumetric energy density and lower Boil-Off Gas losses. As Germany collaborates globally for energy partnerships, the selection of an efficient and sustainable mode of hydrogen transport becomes crucial for realizing the full potential of a hydrogen-based economy.